Update on systemic therapy for colorectal cancer: biologics take sides
Introduction
Fearon and Vogelstein, in their seminal study of a ‘genetic model for colorectal tumorigenesis’ laid out a vision for the deployment of targeted therapeutic agents against alterations associated with the initiation and promotion of colorectal cancer (CRC). They hypothesized that ‘some agents might be sought that would selectively inactivate mutated genes (e.g., ras); others might be obtained that could mimic or restore the normal biologic action of suppressor genes.’ (1). This was an aspirational forecasting of the present era of biologic and targeted therapy. At the time, there was a paucity of effective systemic treatment options, and drug development was focused on traditional chemotherapy. 5-fluorouracil (5FU) modified by leucovorin (LV) delivered significant improvements in overall survival (OS) in the metastatic setting, and the addition of oxaliplatin (OX) as FOLFOX or irinotecan (IRI) as IFL/FOLFIRI to this base also resulted in a doubling of the OS benefit (20 months) compared to 5FU/LV which had been associated with an OS of 11 months (2-4). More recent clinical trials of the triple regimen of active agents - FOLFOXIRI have only shown a marginal improvement in OS, with a tradeoff of increased toxicity (5,6). It is clear that development of chemotherapy for CRC has reached a plateau. On the other hand, biologic targeted therapy development is in ascent and the peak is still far from sight.
The development of targeted therapies in CRC kicked off just after the turn of the 21st century with the investigation of monoclonal antibodies (Moab) targeted at vascular endothelial growth factor (VEGF) and the epidermal growth factor receptor (EGFR). Major improvements in our understanding of immunology led to another class of Moab targeting the immune microenvironment. Antiangiogenic agents (bevacizumab, ramucirumab, ziv-aflibercept and regorafenib) and immune check point inhibitors (pembrolizumab, nivolumab and ipilimumab) are now licensed for the management of metastatic CRC. The benefit of the addition of biologic therapy; specifically, EGFR Moab and antiangiogenic agents to chemotherapy is modest but real and a median OS of between 29 and 36 months is now expected with metastatic CRC (7,8). Accordingly, efforts to develop novel agents for other vulnerabilities in CRC, and to better define factors that predict response to currently available biologics continue. The aim of this review is to provide an update on recent clinically relevant advances in the development of biologic therapy for CRC, with a focus on biomarkers guiding the use of these agents.
Immunotherapy finds a niche
May your road be rough: immune checkpoint inhibitors to the rescue
The classic adenoma-carcinoma sequence, with multistep accumulation of genomic alterations in APC, RAS and DCC is a useful model for conceptualizing CRC development, the majority of which arises from a premalignant polyp. However, 2–3% of patients with hereditary non-polyposis colorectal cancer (HNPCC)/Lynch syndrome do not fall into this category. HNPCC is characterized by microsatellite instability; a by-product of germline mutations in genes that encode mismatch repair (MMR) proteins—MLH1, MSH2, MSH6 and PMS2. Furthermore, 15–20% of patients with sporadic CRC (depending on stage) display a microsatellite instability profile, secondary mainly to hypermethylation of MLH1. Microsatellites are regions of the genome with multiple short DNA repeats 15–25 bp long. These repeats are prone to deletion/insertion errors and frameshift mutations during DNA replication. These errors are corrected by DNA polymerase activity and the MMR proteins. Defective DNA repair therefore allows accumulation of deleterious DNA mutations and subsequent CRC initiation and progression.
The other feature of MMR deficient (MMR-D) CRC pertinent to this discussion is the presence of a lymphocyte-rich tumor infiltrate. Tumor infiltrating lymphocytes (TIL) are part of an immune response to the relatively high burden of neoantigens that occur presumably because of multiple errors in cells with MMR-D (9). In contrast to MMR proficient (MMR-P) CRC with a microsatellite stable (MSS) or microsatellite instability-low (MSI-L) phenotype, the TIL in MMR-D, microsatellite instability high (MSI-H) CRC have an activated profile (10). Interestingly, cytotoxic CD8+ T cells from the peripheral blood of unaffected family members of patients with HNPCC were active against CRC cells (11). Despite this tumor microenvironment that may foster tumor control and better outcomes in early stages, prognosis is poor in patients with more advanced and metastatic MSI-H CRC (12-14). The progression of CRC in this immunogenic background therefore points to the success of immune evasive mechanisms in MSI-H CRC. One evasive maneuver of these tumors is the upregulation of immune check-point inhibitors, including the programmed death ligand-1 (PDL-1) on tumor cells and TIL, which can dampen the activity of activated T-cells (15,16).
Based on the above, it is clear why microsatellite instability would be a logical predictive biomarker for immune check point inhibitor therapy (immunotherapy) in CRC. In an early pilot study of ipilimumab (an anti-CTLA4 monoclonal antibody) in treatment refractory malignancies, there was no response among the 3 patients with CRC (17). Pembrolizumab, an anti-PD-1 monoclonal antibody did not fare better, with no response among 18 unselected CRC patients (18). The KEYNOTE-028 trial was a phase Ib trial designed to assess the safety of pembrolizumab in up to 20 different cohorts of patients with advanced solid tumors. This trial in addition explored the role of PDL-1 as a predictive biomarker for immunotherapy. In the CRC group, 24% of 137 patients’ tumor samples were positive for PD-L1 expression by immunohistochemistry (membrane staining in at least 1% of scorable cells). There was only 1 partial response (PR) among 23 patients with PD-L1 positive CRC (ORR of 4%) treated with pembrolizumab. This response was prolonged, lasting more than 2 years. This patient had MSI-H phenotype and investigators hypothesized that this was a predictive marker for response (19). Simultaneously (and based on the hypothesis that MMR-D CRC would more likely respond to checkpoint inhibition), Le and colleagues recruited 41 patients with metastatic cancer; 11 with MMR-D CRC, 21 with MMR-P CRC and 9 with MMR-D non-CRC. They reported an ORR of 40% in the patients with MMR-D CRC and 0% for MRR-P CRC. The ORR of 71% reported for MMR-D non-colorectal tumors was similar to the number for MMR-D CRC, supporting the role of MMR status as a predictive marker of response to immunotherapy regardless of tumor histology. In addition, pembrolizumab treatment was associated with a disease control rate (DCR) of 90%, and after a median follow up of 36 weeks, median duration of response had not yet been reached in MMR-D patients (20). This study (combined with others) led to the approval of pembrolizumab in the 2nd line for MSI-H CRC, and also contributed to the ‘tumor agnostic’ approval for pembrolizumab in MMR-D/MSI-H malignancies (21). The development of nivolumab, another anti PD-1 antibody in metastatic CRC followed a similar path (22-24). In addition, following the trend of immunotherapy trials, the additive/synergistic effect of anti-PD1 and anti CTLA-4 therapy has been tested in MSI-H CRC. In the CheckMate-142 trial, 119 patients with MMR-D/MSI-H metastatic CRC cancer received the combination of nivolumab and ipilimumab. The ORR was 55% and median progression free survival (PFS) was 71% at 12 months. The OS rate at 12 months was 85% which is quite impressive in a cohort where 76% of patients had received at least 2 lines of systemic therapy (25). This has also led to the approval of nivolumab and ipilimumab for MSI-H CRC in the 2nd line setting. An important concern with this combination is the severity of immune related toxicities especially colitis. Although majority of the patients (73%) had an adverse event (AE), Grade 3 and 4 toxicities were reported in 27% and 5% of patients respectively. Only 3% of patients had grade 3–4 colitis. Among 16 patients with treatment related adverse events (TRAE) leading to discontinuation of therapy, efficacy results were not different from the overall population.
A potential criticism of the approach to the development and licensing of immune check point inhibitors in CRC is the lack of a control arm and a relatively short follow-up period for OS. While randomization and blinding are the gold-standard for clinical trial design, the limitations of this approach for small numbers of patients with a particular biomarker to drive drug development needs to be recognized. The impact of a pragmatic trial design that compares patients with MSI-H CRC to those with MSS CRC and yields impressive response rates and PFS cannot be minimized. Efforts for multi-institutional and international collaborations to allow relatively large randomized trials should continue apace those focused on further delineating new biomarkers for response to immunotherapy in CRC, especially in MSS CRC.
Other biomarkers: tumor mutation burden (TMB) and DNA polymerase mutations
Using microsatellite instability as a predictive biomarker for immunotherapy in CRC limits the use of these agents to less than 5% of the metastatic CRC population, and investments to uncover other important biomarkers are critical. MMR deficiency is one of several mechanisms that may contribute to genomic instability, and accumulation of tumor mutations that foster an immune response to cancer antigens. Ultraviolet (UV) radiation and cigarette smoking cause genomic instability and a high TMB in melanoma and non-small cell lung cancer respectively. Chalmers et al. explored the correlation between TMB and microsatellite instability across cancer types. Based on analysis of about 62,000 samples, they found that MSI-H cancers were a subset of high TMB cancers (defined as 20 non-synonymous mutations/megabase). In that analysis, 83% of MSI-H samples had a high TMB, while only 16% of high TMB samples displayed microsatellite instability (26).
It has thus been hypothesized that it is high TMB, regardless of the underlying cause, that facilitates an immune response that can be augmented/activated by immunotherapy. In melanoma, >100 non-synonymous mutations per exome was associated with an improved RR and survival in response to anti-CTLA4 therapy, and similar findings have been described with anti-PD1 therapy in NSCLC (27,28).
Only few studies have evaluated the role of TMB specifically in CRC (when separated from MMR-D). A retrospective analysis of patients’ samples from the Quick and Simple and Reliable 2 (QUASAR 2) trial of patients with high-risk stage II and III CRC suggested that independent of microsatellite instability, TMB was associated with OS (29). The use of TMB as a biomarker in MSS CRC, so far has been based on extrapolation of data from the melanoma and NSCLC literature. There have been case reports of prolonged response to immunotherapy in this scenario, making further investigation necessary (30,31). Twenty-three percent (of 30 patient samples) with ‘hypermutated’ CRC analyzed in the cancer genome atlas (TCGA) did not have microsatellite instability, and about 3% (of 5,702) MSS CRC samples had a high TMB in another analysis (31,32). Interestingly, alterations in DNA polymerase (POLE)—and a failure of its proofreading function—are the apparent cause of many of these MSS/high TMB cases. DNA polymerase alterations are present in 1–2% of CRC, and there are suggestions that these alterations may be a marker of poor prognosis but improved immune response, similar to MMR deficiency (33). Given the small numbers of patients with MSS and high TMB, it will be difficult to perform large studies to determine the veracity of TMB and DNA polymerase mutations as bona fide predictive biomarkers markers in MSS CRC. However, the growing adoption of next generation sequencing in clinical practice may allow collaborative efforts to pool individual data that may shed more light.
The future remains bright for immunotherapy in CRC with active trials ongoing to establish the role of immunotherapy in the first line either as a stand-alone modality or in combination with chemotherapy. Given the very good prognosis of patients with stage II MSI-H CRC, it is unlikely that immunotherapy would provide a clinically meaningful benefit in the adjuvant setting in this cohort. Already, a pilot study has reported a 100% pathologic complete response (pCR) to neoadjuvant immunotherapy in stage II MSI-H CRC and there is now an ongoing randomized study exploring immunotherapy in addition to chemotherapy for stage III MSI-H CRC. The big challenge is to expand immunotherapy in metastatic MSS CRC, and we anticipate that more work will continue to be done to achieve this goal.
Antiangiogenic and EGFR therapy: extended ras testing and the tale of two colons
Extended ras testing
Cetuximab and panitumumab are the two anti-EGFR monoclonal antibodies (EGFR Moab) approved for use in metastatic CRC. Following initial development for EGFR expressing CRC (which turned out not to be predictive), their use was restricted to Kirsten Rous Sarcoma Virus wild type (KRAS WT) CRC as it became clear that a KRAS mutation (KRAS MT) was associated with lack of response to this class of therapy. In an analysis of patients enrolled into the CO.17 study of cetuximab vs. best supportive care, among 198 patients who received cetuximab, only 1 of the 81 patients (1.2%) with KRAS MT CRC responded to cetuximab compared to a 12.8% ORR among 117 patients with KRAS WT CRC. Furthermore, there was improvement in both PFS (3.7 vs. 1.8 months, P<0.001) and OS (9.5 vs. 4.5 months, P=0.01) in KRAS WT CRC compared to KRAS MT CRC. Correspondingly, there was no significant difference in PFS (1.8 months, HR 0.99, 95% CI: 0.73–1.35, P=0.96) or OS (4.5 vs. 4.6 months, HR 0.98, 95% CI: 0.7–1.37, P=0.89) between cetuximab and BSC in KRAS MT CRC (34). Similar outcomes were reported with panitumumab (35), and these analyses led to an American Society of Clinical Oncology recommendation for KRAS testing prior to administration of EGFR Moab therapy and the restriction treatment to patients with KRAS WT CRC (36).
Initial studies in this domain focused on KRAS exon 2 (codon 13 and 14) mutations, which are the most common KRAS mutations, present in approximately 40% of metastatic CRC. Considering the low response (10–20%) to EGFR Moab therapy eve. in KRAS WT CRC, it is clear that a substantial number of patients with CRC were still being exposed to potentially ineffective therapy. Efforts to uncover more biomarkers that may predict response (or a lack thereof) to EGFR Moab have continued to focus on the EGFR signaling pathway (37,38). Among 60 pre-treated patients with supposedly KRAS exon 2 WT CRC, Andre and colleagues examined less common mutations in exon 3 (codon 59 and 61) KRAS mutations in 6.6% (4 patients) of samples analyzed. They also reported 5 NRAS exon 2 and 3 mutations (8.3%) and 4 BRAF V600E mutations (4.4%). In all, they identified 19 patients with KRAS (including 6 with exon 2, codon 12 mutations), NRAS and BRAF mutations, and reported no response to cetuximab and IRI. The ORR to cetuximab was 46.3% among the patients who were wildtype for all the mutations studied (39). These results were validated in a retrospective review of the PRIME study which compared FOLFOX and panitumumab to FOLFOX alone in the first line setting in KRAS exon 2 WT CRC. In this analysis, KRAS testing was extended to include exons 3 and 4, NRAS exons 2, 3 and 4 and BRAF exon 15 (BRAFV600E). This larger analysis uncovered other RAS mutations in 17% of patients (for a total of around 50%). The OS was worse in patients with so-called ‘extended RAS mutations’ and it was concluded that additional RAS mutations were associated with a negative response to panitumumab. In addition, the combination of panitumumab with FOLFOX was associated with worse PFS and OS compared to FOLFOX only in CRC with RAS mutations suggesting that panitumumab may be harmful in this group. BRAF mutation (discussed further below) carried major prognostic significance but did not appear predictive of outcome relative to panitumumab (40). Along similar lines, investigators uncovered an expanded set of ras family mutations in 14% of 460 patients with KRAS exon 2 WT CRC treated with cetuximab and FOLFIRI versus FOLFIRI in the CRYSTAL study. Cetuximab did not provide any additional benefit to chemotherapy in patients with these other mutations (41). Based on these findings, and several systematic reviews of the existing literature, the ASCO in 2015 updated its provisional clinical opinion, and recommended extended ras testing in CRC patients considered for EGFR Moab therapy. At present, this is best accomplished by sequencing the entire KRAS and NRAS genes.
While the impact of RAS mutations on response to EGFR Moab is clear, the results of findings from other proteins involved in EGFR signaling, particularly BRAF and PIK3CA remain controversial. Systematic reviews and meta-analysis have reported association between mutations in these genes and a lack of response to EGFR Moab, but there are no large prospective trials specifically investigating patients with these mutations (42,43). For instance, the BRAF V600E mutation is associated with poor prognosis in metastatic CRC (44), but its role in predicting response to therapy is still unclear. Early studies had suggested a reduced ORR and survival with EGFR Moab in KRAS WT/BRAF MT CRC, but these studies lacked a control arm. Analysis of the larger, randomized CRYSTAL and PRIME studies failed to confirm or rebut these findings but reinforced the poor prognosis associated with BRAF V600E mutation (40,45).
A tale of two colons
The clinical development of biologic therapy targeting angiogenesis has moved in parallel with EGFR Moab development. The FDA approved Bevacizumab for the management of metastatic CRC in 2004, just before cetuximab was approved. Critically, this approval was not biomarker based. Since then, a number of anti-angiogenic biologic agents including monoclonal antibodies (ramucirumab, ziv-aflibercept) and small molecules (regorafenib) have shown activity in metastatic CRC and are used in the 2nd line and beyond in metastatic CRC. To date, there is still no clinically relevant biomarker to guide the use of bevacizumab. Since EGFR Moab is administered exclusively to patients with RAS WT CRC, the natural question to ask was ‘what is the optimal approach to first line biologic therapy in RAS WT CRC?’
The FIRE-3 study was one of several large studies designed to answer this question (7,46). FIRE-3 randomized 600 patients with KRAS (exon 2) WT metastatic CRC to FOLFIRI and cetuximab or FOLFIRI and bevacizumab. The study was unusual for one of its size in that radiographic response was the primary endpoint of the study. In fact, there was no difference in ORR (62% vs. 58%, odds ratio 1.18, 95% CI: 0.85–1.64; P=0.18) or PFS (10 vs. 10. 3 months, HR 1.06, 95% CI: 0.88–1.26; P=0.55) between the 2 groups. However, OS was 28.7 (95% CI: 24.0–36.6) months in the cetuximab arm compared to 25 (95% CI: 22.7–27.6) months with bevacizumab (HR 0.77, 95% CI: 0.62–0.96, P=0.0017). This complimented the findings of a Phase II trial that randomized patients to FOLFOX and panitumumab or bevacizumab in KRAS (exon 2) WT CRC (47). An OS advantage, in the absence of a PFS benefit with EGFR Moab was puzzling especially as the survival curves appeared to separate sometime after the expected end of first-line therapy. CALBG/SWOG was a significantly larger randomized trial (powered for OS), that did not show a difference in PFS (10.5 vs. 10.6 months, HR 0.95, 95% CI: 0.84–1.08; P=0.45) or OS (30 vs. 29.6 months, HR 0.88, 95% CI: 0.77–1.01; P=0.08) with the addition of cetuximab vs. bevacizumab to chemotherapy (FOLFOX or FOLFIRI) (8) in patients who were WT for KRAS (exon 2) mutation.
In any case, deeper exploration of the results of the above studies uncovered one of the more intriguing concepts in metastatic CRC in the last few years, vis the impact of the primary tumor location (PTL) on the response to biologic therapy. The concept of 2 distinct colons has been around for decades (48), founded on the disparate embryologic origins of the colon. The ascending colon up to the proximal two-thirds of the transverse colon arises from the midgut, while the distal third of the transverse colon, up to the rectum are derived from the hindgut endoderm. The two colons also have different blood supplies, and there is significant evidence of distinct microbiome populations between the two colons. In addition, key clinical and biologic features are described in right sided colon cancer (RCC) compared to left sided colon cancer (LCC). In summary, RCC is commoner among women and affects an older population. RCC tumorigenesis is characterized by a CpG island methylator phenotype (CIMP) and development from serrated polyps. Consequently, there is a higher proportion of MSI-high and BRAF mutations. LCC on the other hand is more likely to be associated with chromosomal instability, KRAS, TP53 and SMAD4 mutations, and development following the classical adenoma-carcinoma sequence. The prognostic impact of PTL is also established and although some investigators have questioned the dogma, several studies support the tenet that RCC carries a worse prognosis than LCC (48,49). In a study involving about 17,000 patients with mainly operable colon cancer, patients with RCC were older and were more likely to be women. RCC was associated with a worse OS (OR 1.12) (50). In the metastatic setting, an analysis of 3 studies involving bevacizumab in addition to chemotherapy also showed that LCC was associated with a more favorable prognosis (51).
Post-hoc analysis of several randomized studies has showed an association between PTL and response to EGFR Moab in KRAS WT CRC. In the Phase III CALGB/SWOG study (that again showed similar PFS and OS with chemotherapy and cetuximab or bevacizumab), further analysis revealed a 10-month improvement in OS favoring 1st line cetuximab compared to bevacizumab in LCC. There was no difference in OS between either treatment regimen for RCC. In addition, in a retrospective analysis of 2 1st line cetuximab and chemotherapy studies (CRYSTAL and FIRE-3), patients with right sided KRAS WT RCC did not seem to benefit from the addition of cetuximab, and multivariate analysis revealed a significant interaction between PTL and OS with respect to cetuximab treatment (52).
While the exact reasons for a lack of benefit with EGFR Moab for KRAS WT RCC are still not clear, it may be that EGFR signaling is not an important driver of RCC, and other alterations may be compensating for EGFR signaling blockade. As one example, promoter methylation appears to lead to lower levels of EGFR ligands amphiregulin and epiregulin. By extension, the increased expression of EGFR and its ligands in LCC has been proposed as a reason for EGFR Moab efficacy in this cohort (53). Based on the above, there is a consensus that EGFR Moab should only be offered to the left sided KRAS WT CRC (at least in the first line of therapy). By extension, the clinical decision making on the use of bevacizumab in the first line setting is guided by PTL and RAS mutation status.
Developments in CRC with BRAF mutation: a raft for BRAF
CRC with a BRAF mutation (BRAF MT) represents a distinct clinical entity characterized by CpG island hypermethylation phenotype (CIMP) and development from serrated polyps (54,55). There is significant overlap with RCC and the associated poor prognosis (56). Indeed, the poor prognosis ascribed to RCC is thought to be driven at least in part by the preponderance of aggressive BRAF MT tumors. Mutations in BRAF occur in 10–15% of metastatic CRC, and the most common mutation (>90%) involves an exon 15, T1779A transversion that leads to substitution of glutamic acid for valine (BRAF V600E) in the BRAF kinase domain. This gain of function mutation instigates ligand independent BRAF activation and signaling (57). Because BRAF signals downstream of KRAS, and KRAS MT CRC is associated with resistance to EGFR Moab therapy, it is reasonable to hypothesize that the BRAF V600E mutation would convey a similar association.
This has been difficult to establish, principally because of the small numbers of BRAF MT CRC. However, given the undeniably poor prognosis of this subtype of CRC, significant effort is underway to improve treatment options. The BRAF V600E mutation is present in about 60% of melanoma, and vemurafenib, a potent inhibitor of oncogenic BRAF yielded an ORR of 53% in previously treated melanoma (58,59). Studies of vemurafenib in chemotherapy refractory BRAF MT CRC however failed to replicate these findings. In one attempt, among 21 patients with BRAF V600E CRC, the ORR was only 5% (60). This disappointing result can be explained by the differences in the biology of both tumors. Prahallad et al., in preclinical studies showed a feedback activation of EGFR and compensatory non-MAPK signaling following BRAF inhibition. They postulated that this was not an active resistance mechanism in melanoma as melanoma cells do not express EGFR to a significant degree. Furthermore, they demonstrated synergistic activity of combined EGFR blockade (with erlotinib or cetuximab) and BRAF (V600E) inhibition (61). In support of this hypothesis, Hyman and colleagues reported a better DCR of 73% (1 PR and 18 stable disease) among 27 patients treated with cetuximab and vemurafenib compared to a DCR of 50% (0 PR and 5 stable disease) in 10 patients treated with vemurafenib only (62). Similar results have been described with the combination of vemurafenib and panitumumab and with combined BRAF and MEK inhibition (63,64). Table 1 summarizes the results of single agent and combination therapy approaches to BRAF MT CRC management. Overall, the preliminary results from the BEACON CRC study represent the most exciting developments in this area thus far. In this ongoing phase 3 trial (NCT02928224), patients are being randomized to a triple regimen of cetuximab, encorafenib and binimetinib (a MEK inhibitor) vs. combination cetuximab and encorafenib or IRI (or FOLFIRI) and cetuximab. Of the 29 patients recruited to the safety lead-in for the triplet combination, a complete response was reported in 1 patient and a PR in 11, adding up to an ORR of 41%. In addition, 31% of patients had stable disease that lasted up to 9 months (70).
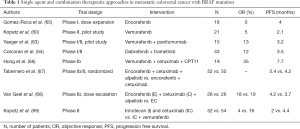
Full table
The activation of PI3K/AKT pathway is another resistance mechanism exploited by BRAF MT CRC both de novo and following exposure to BRAF V600E targeting agents (71). However, a phase Ib study designed to assess the safety of combining alpelisib (a PI3K alpha inhibitor), to cetuximab and encorafenib reported a response rate of 19% (n=28) which was similar to the 18% response rate among 29 patients treated with encorafenib and cetuximab in the same trial (68). This, in addition to the worse AE profile, has dampened the enthusiasm for the development of PI3K inhibitors in this setting. Nevertheless, the encouraging results from the BEACON CRC trial and preclinical work uncovering more resistance mechanisms leave room for optimism that definite progress will be made in the management of BRAF MT CRC in the near future.
Finding a match for HER
As noted in the sections above, abrogating EGFR signaling significantly benefits a select population of patients with metastatic CRC. EGFR belongs to a family of human epidermal growth factor receptor proteins (HER) which also includes HER2, HER3 and HER4. Studies in CRC have focused on the clinical impact of HER2 and HER3 alterations. In contrast to EGFR (HER1) which can be activated by several growth promoting ligands; including amphiregulin, heregulin and other EGF related ligands, HER2 has no known ligands, while HER3 lacks an activating kinase domain. Signal transduction via these receptors proceeds via heterodimerization (HER1/HER2, HER2/HER3) and ligand independent homodimerization (HER2/HER2) in instances of HER2 overexpression. Proliferative and survival signals can therefore be transmitted through these other receptor tyrosine kinases even after EGFR blockade. Accordingly, HER2 (and HER3) overexpression have been suggested as potential de novo resistance and escape mechanisms to EGFR Moab (72,73). For example, in a CRC xenograft model, HER2 overexpression was reported in 18% of KRAS (exon 2) WT CRC who failed to respond to EGFR Moab, whereas none of the 14 cetuximab sensitive KRAS WT CRC showed HER2 overexpression (74). Furthermore, combination treatment with pertuzumab (a humanized IgG1 monoclonal antibody that interferes with HER2 dimerization) and lapatinib (a small molecule EGFR and HER2 tyrosine kinase inhibitor) achieved better tumor control than either agent alone, or in combination with cetuximab in this pre-clinical model. This study supported the hypothesis that HER2 overexpression is a worthy therapeutic target in CRC.
An important challenge in developing HER2 directed targeted therapy is determining the appropriate discriminatory level of HER2 that would allow response to such therapy. Investigators in the HER2 Amplification for Colorectal Cancer Enhanced Stratification (HERACLES) program, formulated consensus criteria for HER2 overexpression in CRC by adapting the criteria already in use in breast and gastric cancer. They defined HER2 overexpression as intense membrane staining of >50% of cells (3+) or ERBB2 gene amplification, captured by in situ hybridization (ISH) at an ERBB2/CEN17 signal ratio equal to or greater than 2 (75). Based on these criteria, HER2 overexpression is reported in 2–3% of unselected CRC with a higher prevalence in the KRAS WT population where the numbers approach 5% (75,76). Investigators in an early phase II trial of trastuzumab and IRI lamented the low prevalence of HER2 in unselected CRC (4%) and identified this as an obstacle to drug development (77). However, given the high prevalence of CRC in the United States and worldwide, the absolute number of appropriately selected patients who may benefit from HER2 targeted therapy is quite substantial and well-designed multi-institutional and international trials are ongoing to move this field forward.
The HERACLES program mentioned above is a multicohort open label Phase II trial of different HER2 targeted therapeutics in chemotherapy and EGFR Moab resistant, RAS WT, HER2 overexpressing CRC. The first cohort testing the combination of trastuzumab and lapatinib showed impressive objective response rate of 30% (1 CR and 7 PR) among 27 enrolled patients. There were no grade 4 or 5 toxicities and grade 3 toxicities reported in 22% of patients were manageable (78). A 2nd cohort, the HERACLES-RESCUE trial is administering T-DM1 (trastuzumab emtasine) to patients who progressed on trastuzumab and lapatinib (NCT03418558). Similarly, in another open-label Phase II basket trial (MyPathway) an ORR of 38% (14 PR) was reported among 37 patients with HER2 overexpressing CRC treated with trastuzumab and pertuzumab (79).
The critical role of HER2 expression as a biomarker for HER2 targeted therapy was highlighted by the UK FOCUS-4D trial. In this randomized phase 2–3 trial, patients with RAS, BRAF and PIK3CA WT CRC were randomized, after stable disease with first-line chemotherapy to maintenance therapy with AZD8931, a pan-HER family kinase inhibitor. HER2 overexpression was not a prerequisite and the trial failed to achieve its primary endpoints. PFS was lower among patients randomized to the trial drug (2.96 vs. 3.48 months, HR 1.1, 95% CI: 0.47–3.57, P=0.95) (80). The HERACLES and MyPathway reports have given rise to justifiable enthusiasm for HER2 targeted therapy in this population. However, it is clear that more work still needs to be done. ORR is around the 30% mark and a substantial proportion of patients are resistant to this therapy. In addition, while current trials are focused on HER2 overexpression, up to 1.5% of unselected CRC may harbor a HER2 mutation (81). It is not clear that the data derived from HER2 overexpressing CRC can be applied to this population. Furthermore, in an ongoing open label Phase II basket trial of neratinib in multiple solid tumors with HER2/3 mutations, there was no response among 12 patients with CRC (NCT01953926). CRC with HER2/3 mutation therefore represents an area for more research.
Future perspectives
In spite of progress that has been made in deploying biologic therapy in CRC, drug development has only so far resulted primarily in defining a target population based on specific alterations rather than targeting the alteration directly (with BRAF and increasingly HER2 perhaps being the exceptions). The latter goal is lofty, but an appreciation of the complex biology of CRC means that clinically relevant druggable driver alterations will occur in only a small proportion of patients with CRC as described with alterations in HER2/3. Arguably, the more clinically impactful challenge is to continue to refine populations that benefit from particular biologic agents (e.g., bevacizumab) while defining novel therapeutic combinations for others like MSS CRC and right sided CRC that are currently underserved with targeted biologic therapy.
Acknowledgements
None.
Footnote
Conflicts of Interest: The authors have no conflicts of interest to declare.
References
- Fearon ER, Vogelstein B. A genetic model for colorectal tumorigenesis. Cell 1990;61:759-67. [Crossref] [PubMed]
- Petrelli N, Herrera L, Rustum Y, et al. A prospective randomized trial of 5-fluorouracil versus 5-fluorouracil and high-dose leucovorin versus 5-fluorouracil and methotrexate in previously untreated patients with advanced colorectal carcinoma. J Clin Oncol 1987;5:1559-65. [Crossref] [PubMed]
- Douillard JY, Cunningham D, Roth AD, et al. Irinotecan combined with fluorouracil compared with fluorouracil alone as first-line treatment for metastatic colorectal cancer: a multicentre randomised trial. Lancet 2000;355:1041-7. [Crossref] [PubMed]
- Giacchetti S, Perpoint B, Zidani R, et al. Phase III multicenter randomized trial of oxaliplatin added to chronomodulated fluorouracil-leucovorin as first-line treatment of metastatic colorectal cancer. J Clin Oncol 2000;18:136-47. [Crossref] [PubMed]
- Falcone A, Ricci S, Brunetti I, et al. Phase III trial of infusional fluorouracil, leucovorin, oxaliplatin, and irinotecan (FOLFOXIRI) compared with infusional fluorouracil, leucovorin, and irinotecan (FOLFIRI) as first-line treatment for metastatic colorectal cancer: the Gruppo Oncologico Nord Ovest. J Clin Oncol 2007;25:1670-6. [Crossref] [PubMed]
- Marques RP, Duarte GS, Sterrantino C, et al. Triplet (FOLFOXIRI) versus doublet (FOLFOX or FOLFIRI) backbone chemotherapy as first-line treatment of metastatic colorectal cancer: A systematic review and meta-analysis. Crit Rev Oncol Hematol 2017;118:54-62. [Crossref] [PubMed]
- Stintzing S, Modest DP, Rossius L, et al. FOLFIRI plus cetuximab versus FOLFIRI plus bevacizumab for metastatic colorectal cancer (FIRE-3): a post-hoc analysis of tumour dynamics in the final RAS wild-type subgroup of this randomised open-label phase 3 trial. Lancet Oncol 2016;17:1426-34. [Crossref] [PubMed]
- Venook AP, Niedzwiecki D, Lenz HJ, et al. Effect of First-Line Chemotherapy Combined With Cetuximab or Bevacizumab on Overall Survival in Patients With KRAS Wild-Type Advanced or Metastatic Colorectal Cancer: A Randomized Clinical Trial. JAMA 2017;317:2392-401. [Crossref] [PubMed]
- Michael-Robinson JM, Biemer-Huttmann A, Purdie DM, et al. Tumour infiltrating lymphocytes and apoptosis are independent features in colorectal cancer stratified according to microsatellite instability status. Gut 2001;48:360-6. [Crossref] [PubMed]
- Phillips SM, Banerjea A, Feakins R, et al. Tumour-infiltrating lymphocytes in colorectal cancer with microsatellite instability are activated and cytotoxic. Br J Surg 2004;91:469-75. [Crossref] [PubMed]
- Schwitalle Y, Kloor M, Eiermann S, et al. Immune response against frameshift-induced neopeptides in HNPCC patients and healthy HNPCC mutation carriers. Gastroenterology 2008;134:988-97. [Crossref] [PubMed]
- Sinicrope FA, Foster NR, Thibodeau SN, et al. DNA mismatch repair status and colon cancer recurrence and survival in clinical trials of 5-fluorouracil-based adjuvant therapy. J Natl Cancer Inst 2011;103:863-75. [Crossref] [PubMed]
- Mohan HM, Ryan E, Balasubramanian I, et al. Microsatellite instability is associated with reduced disease specific survival in stage III colon cancer. Eur J Surg Oncol 2016;42:1680-6. [Crossref] [PubMed]
- Venderbosch S, Nagtegaal ID, Maughan TS, et al. Mismatch repair status and BRAF mutation status in metastatic colorectal cancer patients: a pooled analysis of the CAIRO, CAIRO2, COIN, and FOCUS studies. Clin Cancer Res 2014;20:5322-30. [Crossref] [PubMed]
- Rooney MS, Shukla SA, Wu CJ, et al. Molecular and genetic properties of tumors associated with local immune cytolytic activity. Cell 2015;160:48-61. [Crossref] [PubMed]
- Llosa NJ, Cruise M, Tam A, et al. The vigorous immune microenvironment of microsatellite instable colon cancer is balanced by multiple counter-inhibitory checkpoints. Cancer Discov 2015;5:43-51. [Crossref] [PubMed]
- O’Mahony D, Morris JC, Quinn C, et al. A pilot study of CTLA-4 blockade after cancer vaccine failure in patients with advanced malignancy. Clin Cancer Res 2007;13:958-64. [Crossref] [PubMed]
- Brahmer JR, Tykodi SS, Chow LQ, et al. Safety and activity of anti-PD-L1 antibody in patients with advanced cancer. N Engl J Med 2012;366:2455-65. [Crossref] [PubMed]
- O'Neil BH, Wallmark JM, Lorente D, et al. Safety and antitumor activity of the anti-PD-1 antibody pembrolizumab in patients with advanced colorectal carcinoma. PLoS One 2017;12:e0189848. [Crossref] [PubMed]
- Le DT, Uram JN, Wang H, et al. PD-1 Blockade in Tumors with Mismatch-Repair Deficiency. N Engl J Med 2015;372:2509-20. [Crossref] [PubMed]
- Le DT, Durham JN, Smith KN, et al. Mismatch repair deficiency predicts response of solid tumors to PD-1 blockade. Science 2017;357:409-13. [Crossref] [PubMed]
- Topalian SL, Hodi FS, Brahmer JR, et al. Safety, activity, and immune correlates of anti-PD-1 antibody in cancer. N Engl J Med 2012;366:2443-54. [Crossref] [PubMed]
- Lipson EJ, Sharfman WH, Drake CG, et al. Durable cancer regression off-treatment and effective reinduction therapy with an anti-PD-1 antibody. Clin Cancer Res 2013;19:462-8. [Crossref] [PubMed]
- Overman MJ, McDermott R, Leach JL, et al. Nivolumab in patients with metastatic DNA mismatch repair-deficient or microsatellite instability-high colorectal cancer (CheckMate 142): an open-label, multicentre, phase 2 study. Lancet Oncol 2017;18:1182-91. [Crossref] [PubMed]
- Overman MJ, Lonardi S, Wong KYM, et al. Durable Clinical Benefit With Nivolumab Plus Ipilimumab in DNA Mismatch Repair-Deficient/Microsatellite Instability-High Metastatic Colorectal Cancer. J Clin Oncol 2018;36:773-9. [Crossref] [PubMed]
- Chalmers ZR, Connelly CF, Fabrizio D, et al. Analysis of 100,000 human cancer genomes reveals the landscape of tumor mutational burden. Genome Med 2017;9:34. [Crossref] [PubMed]
- Snyder A, Makarov V, Merghoub T, et al. Genetic basis for clinical response to CTLA-4 blockade in melanoma. N Engl J Med 2014;371:2189-99. [Crossref] [PubMed]
- Rizvi H, Sanchez-Vega F, La K, et al. Molecular Determinants of Response to Anti-Programmed Cell Death (PD)-1 and Anti-Programmed Death-Ligand 1 (PD-L1) Blockade in Patients With Non-Small-Cell Lung Cancer Profiled With Targeted Next-Generation Sequencing. J Clin Oncol 2018;36:633-41. [Crossref] [PubMed]
- Domingo E, Camps C, Kaisaki PJ, et al. Mutation burden and other molecular markers of prognosis in colorectal cancer treated with curative intent: results from the QUASAR 2 clinical trial and an Australian community-based series. Lancet Gastroenterol Hepatol 2018;3:635-43. [Crossref] [PubMed]
- Gong J, Wang C, Lee PP, et al. Response to PD-1 Blockade in Microsatellite Stable Metastatic Colorectal Cancer Harboring a POLE Mutation. J Natl Compr Canc Netw 2017;15:142-7. [Crossref] [PubMed]
- Fabrizio DA, George TJ Jr, Dunne RF, et al. Beyond microsatellite testing: assessment of tumor mutational burden identifies subsets of colorectal cancer who may respond to immune checkpoint inhibition. J Gastrointest Oncol 2018;9:610-7. [Crossref] [PubMed]
- Cancer Genome Atlas N. Comprehensive molecular characterization of human colon and rectal cancer. Nature 2012;487:330-7. [Crossref] [PubMed]
- Domingo E, Freeman-Mills L, Rayner E, et al. Somatic POLE proofreading domain mutation, immune response, and prognosis in colorectal cancer: a retrospective, pooled biomarker study. Lancet Gastroenterol Hepatol 2016;1:207-16. [Crossref] [PubMed]
- Karapetis CS, Khambata-Ford S, Jonker DJ, et al. K-ras mutations and benefit from cetuximab in advanced colorectal cancer. N Engl J Med 2008;359:1757-65. [Crossref] [PubMed]
- Amado RG, Wolf M, Peeters M, et al. Wild-type KRAS is required for panitumumab efficacy in patients with metastatic colorectal cancer. J Clin Oncol 2008;26:1626-34. [Crossref] [PubMed]
- Allegra CJ, Jessup JM, Somerfield MR, et al. American Society of Clinical Oncology provisional clinical opinion: testing for KRAS gene mutations in patients with metastatic colorectal carcinoma to predict response to anti-epidermal growth factor receptor monoclonal antibody therapy. J Clin Oncol 2009;27:2091-6. [Crossref] [PubMed]
- De Roock W, Claes B, Bernasconi D, et al. Effects of KRAS, BRAF, NRAS, and PIK3CA mutations on the efficacy of cetuximab plus chemotherapy in chemotherapy-refractory metastatic colorectal cancer: a retrospective consortium analysis. Lancet Oncol 2010;11:753-62. [Crossref] [PubMed]
- Moroni M, Veronese S, Benvenuti S, et al. Gene copy number for epidermal growth factor receptor (EGFR) and clinical response to antiEGFR treatment in colorectal cancer: a cohort study. Lancet Oncol 2005;6:279-86. [Crossref] [PubMed]
- Andre T, Blons H, Mabro M, et al. Panitumumab combined with irinotecan for patients with KRAS wild-type metastatic colorectal cancer refractory to standard chemotherapy: a GERCOR efficacy, tolerance, and translational molecular study. Ann Oncol 2013;24:412-9. [Crossref] [PubMed]
- Douillard JY, Oliner KS, Siena S, et al. Panitumumab-FOLFOX4 treatment and RAS mutations in colorectal cancer. N Engl J Med 2013;369:1023-34. [Crossref] [PubMed]
- Van Cutsem E, Lenz HJ, Kohne CH, et al. Fluorouracil, leucovorin, and irinotecan plus cetuximab treatment and RAS mutations in colorectal cancer. J Clin Oncol 2015;33:692-700. [Crossref] [PubMed]
- Therkildsen C, Bergmann TK, Henrichsen-Schnack T, et al. The predictive value of KRAS, NRAS, BRAF, PIK3CA and PTEN for anti-EGFR treatment in metastatic colorectal cancer: A systematic review and meta-analysis. Acta Oncol 2014;53:852-64. [Crossref] [PubMed]
- Yang ZY, Wu XY, Huang YF, et al. Promising biomarkers for predicting the outcomes of patients with KRAS wild-type metastatic colorectal cancer treated with anti-epidermal growth factor receptor monoclonal antibodies: a systematic review with meta-analysis. Int J Cancer 2013;133:1914-25. [Crossref] [PubMed]
- Richman SD, Seymour MT, Chambers P, et al. KRAS and BRAF mutations in advanced colorectal cancer are associated with poor prognosis but do not preclude benefit from oxaliplatin or irinotecan: results from the MRC FOCUS trial. J Clin Oncol 2009;27:5931-7. [Crossref] [PubMed]
- Van Cutsem E, Kohne CH, Lang I, et al. Cetuximab plus irinotecan, fluorouracil, and leucovorin as first-line treatment for metastatic colorectal cancer: updated analysis of overall survival according to tumor KRAS and BRAF mutation status. J Clin Oncol 2011;29:2011-9. [Crossref] [PubMed]
- Heinemann V, von Weikersthal LF, Decker T, et al. FOLFIRI plus cetuximab versus FOLFIRI plus bevacizumab as first-line treatment for patients with metastatic colorectal cancer (FIRE-3): a randomised, open-label, phase 3 trial. Lancet Oncol 2014;15:1065-75. [Crossref] [PubMed]
- Schwartzberg LS, Rivera F, Karthaus M, et al. PEAK: a randomized, multicenter phase II study of panitumumab plus modified fluorouracil, leucovorin, and oxaliplatin (mFOLFOX6) or bevacizumab plus mFOLFOX6 in patients with previously untreated, unresectable, wild-type KRAS exon 2 metastatic colorectal cancer. J Clin Oncol 2014;32:2240-7. [Crossref] [PubMed]
- Bufill JA. Colorectal cancer: evidence for distinct genetic categories based on proximal or distal tumor location. Ann Intern Med 1990;113:779-88. [Crossref] [PubMed]
- Petrelli F, Tomasello G, Borgonovo K, et al. Prognostic Survival Associated With Left-Sided vs. Right-Sided Colon Cancer: A Systematic Review and Meta-analysis. JAMA Oncol 2016. [PubMed]
- Benedix F, Kube R, Meyer F, et al. Comparison of 17,641 patients with right- and left-sided colon cancer: differences in epidemiology, perioperative course, histology, and survival. Dis Colon Rectum 2010;53:57-64. [Crossref] [PubMed]
- Loupakis F, Yang D, Yau L, et al. Primary tumor location as a prognostic factor in metastatic colorectal cancer. J Natl Cancer Inst 2015.107. [PubMed]
- Tejpar S, Stintzing S, Ciardiello F, et al. Prognostic and Predictive Relevance of Primary Tumor Location in Patients With RAS Wild-Type Metastatic Colorectal Cancer: Retrospective Analyses of the CRYSTAL and FIRE-3 Trials. JAMA Oncol 2016. [PubMed]
- Missiaglia E, Jacobs B, D'Ario G, et al. Distal and proximal colon cancers differ in terms of molecular, pathological, and clinical features. Ann Oncol 2014;25:1995-2001. [Crossref] [PubMed]
- English DR, Young JP, Simpson JA, et al. Ethnicity and risk for colorectal cancers showing somatic BRAF V600E mutation or CpG island methylator phenotype. Cancer Epidemiol Biomarkers Prev 2008;17:1774-80. [Crossref] [PubMed]
- Rad R, Cadinanos J, Rad L, et al. A genetic progression model of Braf(V600E)-induced intestinal tumorigenesis reveals targets for therapeutic intervention. Cancer Cell 2013;24:15-29. [Crossref] [PubMed]
- Clancy C, Burke JP, Kalady MF, et al. BRAF mutation is associated with distinct clinicopathological characteristics in colorectal cancer: a systematic review and meta-analysis. Colorectal Dis 2013;15:e711-8. [Crossref] [PubMed]
- Wan PT, Garnett MJ, Roe SM, et al. Mechanism of activation of the RAF-ERK signaling pathway by oncogenic mutations of B-RAF. Cell 2004;116:855-67. [Crossref] [PubMed]
- Davies H, Bignell GR, Cox C, et al. Mutations of the BRAF gene in human cancer. Nature 2002;417:949-54. [Crossref] [PubMed]
- Sosman JA, Kim KB, Schuchter L, et al. Survival in BRAF V600-mutant advanced melanoma treated with vemurafenib. N Engl J Med 2012;366:707-14. [Crossref] [PubMed]
- Kopetz S, Desai J, Chan E, et al. Phase II Pilot Study of Vemurafenib in Patients With Metastatic BRAF-Mutated Colorectal Cancer. J Clin Oncol 2015;33:4032-8. [Crossref] [PubMed]
- Prahallad A, Sun C, Huang S, et al. Unresponsiveness of colon cancer to BRAF(V600E) inhibition through feedback activation of EGFR. Nature 2012;483:100-3. [Crossref] [PubMed]
- Hyman DM, Puzanov I, Subbiah V, et al. Vemurafenib in Multiple Nonmelanoma Cancers with BRAF V600 Mutations. N Engl J Med 2015;373:726-36. [Crossref] [PubMed]
- Yaeger R, Cercek A, O'Reilly EM, et al. Pilot trial of combined BRAF and EGFR inhibition in BRAF-mutant metastatic colorectal cancer patients. Clin Cancer Res 2015;21:1313-20. [Crossref] [PubMed]
- Corcoran RB, Atreya CE, Falchook GS, et al. Combined BRAF and MEK Inhibition With Dabrafenib and Trametinib in BRAF V600-Mutant Colorectal Cancer. J Clin Oncol 2015;33:4023-31. [Crossref] [PubMed]
- Gomez-Roca CA, Delord J, Hidalgo RM, et al. Encorafenib (LGX818), an oral BRAF inhibitor, in patients (pts) with BRAF V600E metasatic colorectal cancer (mCRC): Results of dose expansion in an open-label, phase 1 study. Ann Oncol 2014;25:iv182-3. [Crossref]
- Hong DS, Morris VK, El Osta B, et al. Phase IB Study of Vemurafenib in Combination with Irinotecan and Cetuximab in Patients with Metastatic Colorectal Cancer with BRAFV600E Mutation. Cancer Discov 2016;6:1352-65. [Crossref] [PubMed]
- Tabernero J, Geel RV, Guren TK, et al. Phase 2 results: Encorafenib (ENCO) and cetuximab (CETUX) with or without alpelisib (ALP) in patients with advanced BRAF-mutant colorectal cancer (BRAFm CRC). J Clin Oncol 2016;34:3544. [Crossref]
- van Geel R, Tabernero J, Elez E, et al. A Phase Ib Dose-Escalation Study of Encorafenib and Cetuximab with or without Alpelisib in Metastatic BRAF-Mutant Colorectal Cancer. Cancer Discov 2017;7:610-9. [Crossref] [PubMed]
- Kopetz S, McDonough SL, Morris VK, et al. Randomized trial of irinotecan and cetuximab with or without vemurafenib in BRAF-mutant metastatic colorectal cancer (SWOG 1406). J Clin Oncol 2017;35:520. [Crossref]
- Cutsem EV, Cuyle P-J, Huijberts S, et al. BEACON CRC study safety lead-in (SLI) in patients with BRAFV600E metastatic colorectal cancer (mCRC): Efficacy and tumor markers. J Clin Oncol 2018;36:627. [Crossref]
- Mao M, Tian F, Mariadason JM, et al. Resistance to BRAF inhibition in BRAF-mutant colon cancer can be overcome with PI3K inhibition or demethylating agents. Clin Cancer Res 2013;19:657-67. [Crossref] [PubMed]
- Scartozzi M, Mandolesi A, Giampieri R, et al. The role of HER-3 expression in the prediction of clinical outcome for advanced colorectal cancer patients receiving irinotecan and cetuximab. Oncologist 2011;16:53-60. [Crossref] [PubMed]
- Wheeler DL, Huang S, Kruser TJ, et al. Mechanisms of acquired resistance to cetuximab: role of HER (ErbB) family members. Oncogene 2008;27:3944-56. [Crossref] [PubMed]
- Bertotti A, Migliardi G, Galimi F, et al. A molecularly annotated platform of patient-derived xenografts (“xenopatients”) identifies HER2 as an effective therapeutic target in cetuximab-resistant colorectal cancer. Cancer Discov 2011;1:508-23. [Crossref] [PubMed]
- Valtorta E, Martino C, Sartore-Bianchi A, et al. Assessment of a HER2 scoring system for colorectal cancer: results from a validation study. Mod Pathol 2015;28:1481-91. [Crossref] [PubMed]
- Richman SD, Southward K, Chambers P, et al. HER2 overexpression and amplification as a potential therapeutic target in colorectal cancer: analysis of 3256 patients enrolled in the QUASAR, FOCUS and PICCOLO colorectal cancer trials. J Pathol 2016;238:562-70. [Crossref] [PubMed]
- Ramanathan RK, Hwang JJ, Zamboni WC, et al. Low overexpression of HER-2/neu in advanced colorectal cancer limits the usefulness of trastuzumab (Herceptin) and irinotecan as therapy. A phase II trial. Cancer Invest 2004;22:858-65. [Crossref] [PubMed]
- Sartore-Bianchi A, Trusolino L, Martino C, et al. Dual-targeted therapy with trastuzumab and lapatinib in treatment-refractory, KRAS codon 12/13 wild-type, HER2-positive metastatic colorectal cancer (HERACLES): a proof-of-concept, multicentre, open-label, phase 2 trial. Lancet Oncol 2016;17:738-46. [Crossref] [PubMed]
- Hainsworth JD, Meric-Bernstam F, Swanton C, et al. Targeted Therapy for Advanced Solid Tumors on the Basis of Molecular Profiles: Results From MyPathway, an Open-Label, Phase IIa Multiple Basket Study. J Clin Oncol 2018;36:536-42. [Crossref] [PubMed]
- Adams R, Brown E, Brown L, et al. Inhibition of EGFR, HER2, and HER3 signalling in patients with colorectal cancer wild-type for BRAF, PIK3CA, KRAS, and NRAS (FOCUS4-D): a phase 2-3 randomised trial. Lancet Gastroenterol Hepatol 2018;3:162-71. [Crossref] [PubMed]
- Ross JS, Fakih M, Ali SM, et al. Targeting HER2 in colorectal cancer: The landscape of amplification and short variant mutations in ERBB2 and ERBB3. Cancer 2018;124:1358-73. [Crossref] [PubMed]
Cite this article as: Gbolahan O, O’Neil B. Update on systemic therapy for colorectal cancer: biologics take sides. Transl Gastroenterol Hepatol 2019;4:9.