Glypican-1 exosomes: do they initiate a new era for early pancreatic cancer diagnosis?
According to the National and Comprehensive Cancer Network (NCCN), currently pancreatic cancer (PC) is the fourth leading cause of cancer-related death among US males and females (1). Peak incidence of PC is around the 7th–8th decade of life, and it has been linked to few predisposing factors including: cigarette smoking, obesity, red meat and heavy alcohol consumption along with chronic pancreatitis (1).
Because of its aggressive behavior and frequently late diagnosis, research aiming to early identification of PC or its precursors, could be crucial.
In a recent paper by Melo and associates, authors identified circulating glypican-1 (GPC1) exosomes as a peculiar hallmark of PC, useful also for identifying the early disease (2).
The aim of this commentary is to explore and highlight the Nature publishing authors’ findings, starting with a brief introduction focused on the research background.
Glypican-1 (GPC1) and pancreatic cancer (PC)
Glypicans constitute a family of heparan sulfate proteoglycans (HSPGs) that are connected to the exocytoplasmic domain of the cell membrane by a glycosyl-phosphatidylinositol (GPI) anchor, and, to-date, several members of the glypican family, specifically six in mammals (GPC1–GPC6) and two in Drosophila, have been identified (3,4) (Table 1).
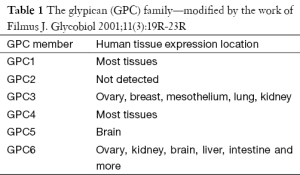
Full table
Reports connecting GPC1 associated to PC begin almost 15 years ago, when Kleeff and co-authors documented that GPC1 is over-expressed in human PC and in adjacent stroma fibroblasts, its antisense strikingly decreases the tumorigenicity of PC cells, and GPC1 itself plays an important role in the response to several mitogenic stimuli, such as HB-EGF (5,6) and FGF-2 (5-7).
Moreover, the same group later documented that in PC cells, GPC1 is required for an efficient TGF-β1 signaling (8). It was later shown that GPC1 down-regulation suppresses PC cell growth and slightly modifies signaling of members of the TGF-β family (9).
Exosomes
Extracellular vesicles (EV) family includes exosomes, microvesicles and a variety of vesicles released by many cellular types (10-12).
Literature reports a growing body of evidence suggesting that cancer cells release more EV than normal ones, partly in relation to the response of a number of oncogenes, including e.g., ras (2,13-16).
The term exosome was firstly used in 1981 to describe membrane-enclosed structures of 40 to 500–1,000 nm in diameter that had been ‘exfoliated’ from the surface of cultured cells (17). The same term was proposed in 1987 for 50–100 nm vesicles that form inside endosomes, and that are released in the extracellular compartment when endosomes fuse with the cellular membrane (18). Opposite, microvesicles are >1,000 nm and bud directly from plasma membrane (19).
EVs circulate in the blood, urine, ascites, and cerebrospinal fluid (20-23), also in cancer patients: indeed, circulating EVs have been detected in the blood samples of patients with glioblastoma, colorectal, ovarian and non-small cell (NSC) lung cancer (24-27).
Analysis of exosomes could be highly significant, since they contain proteins, nucleic acids (coding- and non-coding RNAs and DNAs), thus it can provide information on the primary tumor and along with its microenvironment (28-30).
The main functions of exosomes in cancer microenvironment are to promote cancer growth, to stimulate the angiogenesis, to activate stromal fibroblasts, to sculpture cancer extracellular matrix, to generate pre-metastatic niche and to suppress the host immune responses (31).
Their laboratory detection could be, however, challenging, mostly due to their small size that often involves several ultra-centrifugations cycles, in order to concentrate a large volume and amount of material. To address these technological challenges, a series of miniaturized systems have been developed to facilitate exosome analyses including micro-fluidic enrichment and magnetic detection (32).
Circulating glypican-1 (GPC1) exosomes in pancreatic cancer (PC)
Melo and co-authors recently reported the detection of circulating exosomes in breast, PC patients, as well as healthy donors (2). The investigation required several lab-bases analyses, as isolation of EVs by ultra-centrifugation, NanoSight nanoparticle tracking and transmission electron microscopy (TEM); proteins were evaluated by ultra-performance liquid chromatography-mass spectrometry (UPLC-MS) and GPC1 was detected exclusively in cancer exosomes using immunogold-TEM and immunoblot.
This case-control translational study revealed that the overall concentration of exosomes in cancer patients and the concentration of circulating GPC1 EVs in PCs were significantly higher comparing controls. Furthermore, circulating GPC1 EVs of PC patients harbor K-ras mutations in 65.9% of the cases (31 out of 47 PC analyzed) (Figure 1).
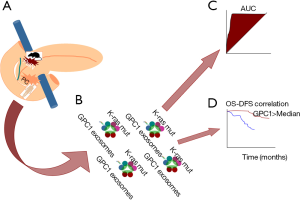
On the same extent, the main and innovative finding reported in this paper was the striking perfection of ROC curve analysis when analyzing the concentration of GPC1 exosomes for distinguishing cancer from benign diseases and cancer in situ from advantages stages in a clinical series of patients, as well as pre-malignant lesions (PanIN) from PC in a mice model (AUC 1.0).
Of note, neither the size nor the overall concentration of circulating exosomes were documented valid, and also Ca19.9 was proven inferior comparing GPC1 exosomes, suggesting these latter as a peculiar hallmark of PC, suitable also as candidates for early detection.
The primary application could be screening of PanIN patients, although human data should be advocated. It has been documented that K-ras are the driver mutations for PC and are detected in early PanIN-1, but it requires 15–20 years for this lesion to progress into metastatic PC (33-35), thus un-invasive monitoring of these patients could be extremely useful. Other translational uses could be the screening of circulating GPC1 exosomes in selected risk categories of patients, as alcoholics, obese, chronic pancreatitis or patients presenting a familial risk.
Another important clinical use could be the prediction of survival of PC patients, indeed Melo and associates, using a Cox multivariate regression analysis documented that the decrease of circulating GPC1 exosomes as an independent prognostic marker predictive for disease-specific survival. This result confirms previous finding by Duan and co-authors, who reported that GPC1 is significantly related to the perineural invasion in PC and it has an independent prognostic effect on the overall survivals (36).
Conclusions
Undoubtedly Melo findings should be confirmed in larger series of patients, but the striking laboratory evidences provided in this paper could open and promote (from bench to bed) a new prospective era for early PC detection.
Acknowledgements
None.
Footnote
Conflicts of Interest: The authors have no conflicts of interest to declare.
References
- NCCN Guidelines, Pancreatic adenocarcinoma, version 2.2015. Available online: www.nccn.org
- Melo SA, Luecke LB, Kahlert C, et al. Glypican-1 identifies cancer exosomes and detects early pancreatic cancer. Nature 2015;523:177-82. [Crossref] [PubMed]
- Nakato H, Futch TA, Selleck SB. The division abnormally delayed (dally) gene: a putative integral membrane proteoglycan required for cell division patterning during postembryonic development of the nervous system in Drosophila. Development 1995;121:3687-702. [PubMed]
- Baeg GH, Lin X, Khare N, et al. Heparan sulfate proteoglycans are critical for the organization of the extracellular distribution of Wingless. Development 2001;128:87-94. [PubMed]
- Kleeff J, Ishiwata T, Kumbasar A, et al. The cell-surface heparan sulfate proteoglycan glypican-1 regulates growth factor action in pancreatic carcinoma cells and is overexpressed in human pancreatic cancer. J Clin Invest 1998;102:1662-73. [Crossref] [PubMed]
- Kleeff J, Wildi S, Kumbasar A, et al. Stable transfection of a glypican-1 antisense construct decreases tumorigenicity in PANC-1 pancreatic carcinoma cells. Pancreas 1999;19:281-8. [Crossref] [PubMed]
- Ding K, Lopez-Burks M, Sánchez-Duran JA, et al. Growth factor-induced shedding of syndecan-1 confers glypican-1 dependence on mitogenic responses of cancer cells. J Cell Biol 2005;171:729-38. [Crossref] [PubMed]
- Li J, Kleeff J, Kayed H, et al. Glypican-1 antisense transfection modulates TGF-beta-dependent signaling in Colo-357 pancreatic cancer cells. Biochem Biophys Res Commun 2004;320:1148-55. [Crossref] [PubMed]
- Kayed H, Kleeff J, Keleg S, et al. Correlation of glypican-1 expression with TGF-beta, BMP, and activin receptors in pancreatic ductal adenocarcinoma. Int J Oncol 2006;29:1139-48. [PubMed]
- van der Vlist EJ, Nolte-'t Hoen EN, Stoorvogel W, et al. Fluorescent labeling of nano-sized vesicles released by cells and subsequent quantitative and qualitative analysis by high-resolution flow cytometry. Nat Protoc 2012;7:1311-26. [Crossref] [PubMed]
- Raposo G, Stoorvogel W. Extracellular vesicles: exosomes, microvesicles, and friends. J Cell Biol 2013;200:373-83. [PubMed]
- Gouveia de Andrade AV, Bertolino G, Riewaldt J, et al. Extracellular vesicles secreted by bone marrow- and adipose tissue-derived mesenchymal stromal cells fail to suppress lymphocyte proliferation. Stem Cells Dev 2015;24:1374-6. [Crossref] [PubMed]
- Mytar B, Baj-Krzyworzeka M, Majka M, et al. Human monocytes both enhance and inhibit the growth of human pancreatic cancer in SCID mice. Anticancer Res 2008;28:187-92. [PubMed]
- Ratajczak J, Miekus K, Kucia M, et al. Embryonic stem cell-derived microvesicles reprogram hematopoietic progenitors: evidence for horizontal transfer of mRNA and protein delivery. Leukemia 2006;20:847-56. [Crossref] [PubMed]
- Kim HK, Song KS, Park YS, et al. Elevated levels of circulating platelet microparticles, VEGF, IL-6 and RANTES in patients with gastric cancer: possible role of a metastasis predictor. Eur J Cancer 2003;39:184-91. [Crossref] [PubMed]
- Rechavi O, Goldstein I, Kloog Y. Intercellular exchange of proteins: the immune cell habit of sharing. FEBS Lett 2009;583:1792-9. [Crossref] [PubMed]
- Trams EG, Lauter CJ, Salem N Jr, et al. Exfoliation of membrane ecto-enzymes in the form of micro-vesicles. Biochim Biophys Acta 1981;645:63-70. [Crossref] [PubMed]
- Johnstone RM, Adam M, Hammond JR, et al. Vesicle formation during reticulocyte maturation. Association of plasma membrane activities with released vesicles (exosomes). J Biol Chem 1987;262:9412-20. [PubMed]
- Qian Z, Shen Q, Yang X, et al. The Role of Extracellular Vesicles: An Epigenetic View of the Cancer Microenvironment. Biomed Res Int 2015;2015:649161.
- Graves LE, Ariztia EV, Navari JR, et al. Proinvasive properties of ovarian cancer ascites-derived membrane vesicles. Cancer Res 2004;64:7045-9. [Crossref] [PubMed]
- Piccin A, Murphy WG, Smith OP. Circulating microparticles: pathophysiology and clinical implications. Blood Rev 2007;21:157-71. [Crossref] [PubMed]
- Smalley DM, Sheman NE, Nelson K, et al. Isolation and identification of potential urinary microparticle biomarkers of bladder cancer. J Proteome Res 2008;7:2088-96. [Crossref] [PubMed]
- Taylor DD, Gercel-Taylor C. MicroRNA signatures of tumor-derived exosomes as diagnostic biomarkers of ovarian cancer. Gynecol Oncol 2008;110:13-21. [Crossref] [PubMed]
- Shao H, Chung J, Balaj L, et al. Protein typing of circulating microvesicles allows real-time monitoring of glioblastoma therapy. Nat Med 2012;18:1835-40. [Crossref] [PubMed]
- Yoshioka Y, Kosaka N, Konishi Y, et al. Ultra-sensitive liquid biopsy of circulating extracellular vesicles using ExoScreen. Nat Commun 2014;5:3591. [Crossref] [PubMed]
- Im H, Shao H, Park YI, et al. Label-free detection and molecular profiling of exosomes with a nano-plasmonic sensor. Nat Biotechnol 2014;32:490-5. [Crossref] [PubMed]
- Frydrychowicz M, Kolecka-Bednarczyk A, Madejczyk M, et al. Exosomes - structure, biogenesis and biological role in non-small-cell lung cancer. Scand J Immunol 2015;81:2-10. [Crossref] [PubMed]
- Valadi H, Ekström K, Bossios A, et al. Exosome-mediated transfer of mRNAs and microRNAs is a novel mechanism of genetic exchange between cells. Nat Cell Biol 2007;9:654-9. [Crossref] [PubMed]
- Skog J, Würdinger T, van Rijn S, et al. Glioblastoma microvesicles transport RNA and proteins that promote tumour growth and provide diagnostic biomarkers. Nat Cell Biol 2008;10:1470-6. [Crossref] [PubMed]
- Balaj L, Lessard R, Dai L, et al. Tumour microvesicles contain retrotransposon elements and amplified oncogene sequences. Nat Commun 2011;2:180. [Crossref] [PubMed]
- Ge R, Tan E, Sharghi-Namini S, et al. Exosomes in Cancer Microenvironment and Beyond: have we Overlooked these Extracellular Messengers? Cancer Microenviron 2012;5:323-32. [Crossref] [PubMed]
- Shao H, Chung J, Issadore D. Diagnostic technologies for circulating tumor cells and exosomes. Biosci Rep 2015;36:e00292. [Crossref] [PubMed]
- Murphy SJ, Hart SN, Lima JF, et al. Genetic alterations associated with progression from pancreatic intraepithelial neoplasia to invasive pancreatic tumor. Gastroenterology 2013;145:1098-1109.e1.
- Bardeesy N, DePinho RA. Pancreatic cancer biology and genetics. Nat Rev Cancer 2002;2:897-909. [Crossref] [PubMed]
- Yachida S, Jones S, Bozic I, et al. Distant metastasis occurs late during the genetic evolution of pancreatic cancer. Nature 2010;467:1114-7. [Crossref] [PubMed]
- Duan L, Hu XQ, Feng DY, et al. GPC-1 may serve as a predictor of perineural invasion and a prognosticator of survival in pancreatic cancer. Asian J Surg 2013;36:7-12. [Crossref] [PubMed]
Cite this article as: Lorenzon L, Blandino G. Glypican-1 exosomes: do they initiate a new era for early pancreatic cancer diagnosis? Transl Gastroenterol Hepatol 2016;1:8.